VERMICOMPOSTING EXPERIMENT REPORT
Jesse Simko
March 2000
Abstract
A vermicomposting experiment was conducted over a nine week period in an attempt to answer several questions on topics such as the effects of earthworms upon decomposing organic materials, shifts in compost weight, depth, and density, and the relative decomposition rates of various organic materials. Several types of compost were analyzed to determine which composting methods most successfully produce usable soil. It was found that food scraps decompose more quickly than leaves and lose more weight and volume in the process. The inclusion of worms had no significant effect upon the decomposition process in this experiment. Much was learned about the factors that contribute to a successful compost, and the conditions necessary for worms to survive.
Introduction
For this experiment, six different compost treatments were studied over a nine week period in an attempt to answer several questions. The main objective was to determine which of six different composting treatments produced the best humus, or fertile soil. The treatments consisted of different combinations of food scraps, leaves, and worms. An important question was which compost ingredients would decompose more quickly: leaves, or food scraps? It was expected that food scraps would decompose more quickly than leaves, for the simple reason that they would be more quickly processed by bacteria and worms. Assuming that food scraps did indeed decompose more quickly than leaves, could the decomposition rate of the leaves be increased by adding food to the leaves, or does food decompose independently, without having an effect on the decomposition rate of nearby leaves? Also, would the amount of input (food, leaves, and worms) equal the amount of output (fertile compost), or would biomass be lost during the composting process? What might cause the compost bins to lose weight during the experiment? How much do the organic contents of a compost bin settle during the composting process, and how would changes in the mass and depth of the compost affect the density of the finished product? A final point of interest was the effect of worms upon the decomposition process. Could the presence of worms speed up the process, or is the work of the worms greatly outweighed by that of unseen microbes? What effects might worms have upon the weight and depth of a compost? Also, would worms thrive in the presence of rotting food? How would they fare in a compost full of rotting leaves? This experiment attempted to answer all of these questions.
Background Information and Literature Review
Much is already known about the biological process of composting. The decomposition, or stabilization of organic material can occur in one of two ways: aerobic decomposition, and anaerobic fermentation. (Gottas, 1956.)
In the aerobic composting process, air-breathing microbes consume compost material and convert it into fertile soil. In the early stages of this process, mesophilic microorganisms break down the soluble, most readily degradable parts of the compost. These microbes thrive in a moist environment of a moderate temperature. They consume organic matter, which is used to develop cell protoplasm. This comes mainly from nitrogen and carbon. Other nutrients such as phosphorus and potassium are also needed, but in much smaller amounts. About two-thirds of the carbon that is used by microbes is converted to energy and is burned up and released as carbon dioxide. The other third of the carbon is combined with nitrogen in the microbes' cells. This entire process requires 12-15 times more carbon than nitrogen. (Seekins, 1999.) If the excess of carbon over nitrogen is too great however, several repetitions of the process are necessary before all of the carbon can be burned by the microbes. Once the ratio of carbon to nitrogen is low enough, excess nitrogen is released in the from of ammonia gas. Heat is released as carbon and binds with oxygen to form carbon dioxide. Under the right conditions, enough heat is produced to cause thermophilic (or heat-loving) microorganisms to take over the task of decomposition. This occurs at a temperature of approximately 104 degrees F.
The temperature inside an active compost normally reaches 120 F within one to five days, and it can sometimes reach as high as 150 F. A direct benefit of the high temperature of aerobic compost is the breakdown of proteins, fats, and complex carbohydrates, which provide structural support to plants. These temperatures destroy pathogenic bacteria, protozoa, hookworm eggs, and most importantly, weed seeds. With the elimination of these unwanted pests comes the elimination of undesirable odors. The optimal temperature is slightly above 130 F. Temperatures above 150 F however, can kill even the beneficial microbes. (Gottas 1956.) Eventually the supply of necessary compounds is exhausted by thermophilic microbes. When this happens the compost temperature decreases, allowing mesophilic microorganisms to return to complete the composting task by curing the remaining organic material. For the aerobic decomposition process to work properly, a constant supply of oxygen must be available to the microbes within the compost. Oxygen can be added by simply turning over the compost, exposing it to air.
Moisture is a critical component of this process. Microorganisms thrive when moisture exists on the surface of all the particles in a compost. This serves as a medium to live and move in. Another important function of moisture is to keep nutrients in solution. Microbes can only consume nutrients if they are dissolved in a solution. For example, if there is not enough moisture to keep ammonia in solution, it would become a gas. Soon the compost would smell like ammonia. (Seekins, 1999.)
In some ways, the anaerobic fermentation process is similar to the aerobic decomposition process. In the anaerobic process, microorganisms that do not require oxygen metabolize nutrients from the compost. These organic nutrients are broken down into their component parts. The anaerobic organisms utilize carbon, nitrogen and other elements from the compost to make cell protoplasm. The nitrogen is reduced to a series of organic acids and ammonia. Some of the carbon is used to fuel the cells of the microbes. The rest of the carbon is released in the form of methane. This causes a terrible odor to emit from the compost. Unlike aerobic decomposition, anaerobic fermentation does not release energy as heat. The energy that could have been released as heat is instead retained within the microbes. Unfortunately the cooler temperature within anaerobic compost allows for the proliferation of pathogenic organisms.
In a BioCycle article, Bill Seekens briefly summarizes the differences between the aerobic and anaerobic composting processes:
Composting is predominantly an aerobic or oxygen-requiring process. The microbes need air to function. Even the facultative variety, those organisms that can operate in aerobic and anaerobic modes, should have oxygen if they are going to function in the aerobic mode -- the mode we want. Under aerobic conditions, the primary breakdown products are carbon dioxide and water. In the anaerobic mode, organic acids are produced. This is where a lot of odor problems come from. So we want to provide enough oxygen for all aerobic organisms to prosper, and for facultative organisms to stay in the aerobic mode. (Seekins, 1999.)
Worm Composting
A large amount of information is known about the effects of earthworms on compost. Earthworms are capable of eating their weight in soil and organic material every day. (Thomas, 1999.) Worms generally leave behind compost that is between five and eleven times as rich is the material they consume. Earthworms are capable of this because their intestinal tracts contain microorganisms that separate valuable plant nutrients from unneeded material. Earthworm excrement is full of rich humus. (Thomas, 1999).
Earthworms continue the process of making the soil more rich until there is nothing left but dark, dry humus. Worms thrive as long as their simple needs are met. They must have sufficient oxygen to do their job, meaning they can not operate within an anaerobic compost pile. In addition, they can not tolerate the heat of an active aerobic compost heap. When worms are exposed to high temperatures they melt. This presents a seemingly unsolvable dilemma, since the aerobic composts that worms commonly inhabit heat up to deadly temperatures. There are two solutions to this problem. The first is to use a very shallow compost bin. This increases the surface area of the compost, ensuring that all parts of the compost heap remain close to the cool outside air. The temperature of the compost is reduced greatly, preventing microbes from doing their job, but allowing earthworms to do theirs. Another solution is to use worms only after the heat of the compost has dissipated. About three weeks after the last of the compost ingredients are added, an aerobic compost will return to a normal temperature. Once the compost has returned to a suitable temperature, the worms can be added. under the right conditions, a population of earthworms will grow by an order of 1,000 in as little as one or two years. (Minnach, 1979.)
Methods
For this experiment, twenty-four small composting bins made out of 2-liter plastic soda bottles were set up and filled with different organic materials. The partial transparency of the bottles made it possible to observe the changes that occurred during the course of the experiment. Six different compost treatments, labeled A through F, were tested. Each of the six treatments was repeated four times to ensure accuracy through redundancy and to allow an ANOVA (Analysis of Variance) to be conducted once the final data had been gathered. Different combinations of leaves and organic food products were used in the compost treatments. The leaf portion of the compost was composed of an even mixture of maple and oak leaves. The resulting mixture was cut into small pieces. The food portion was composed of an uneven mixture of lettuce, cabbage, beats, and small amounts of other fresh vegetables. In addition, half of the compost treatments received 50 grams of earthworms. Table 1 shows the quantities of food, leaves, and worms that were added to each of the six compost treatments.
Table 1: quantities of leaves and food, and worms added to each compost bin:
Treatment I.D. |
Leaves (mL) |
Food (mL) |
Worms (g) |
A: Bottles 1-4 |
1500
|
0
|
0
|
B: Bottles 5-8 |
750
|
750
|
0
|
C: Bottle 9-12 |
0
|
1500
|
0
|
D: Bottles 13-16 |
1500
|
0
|
50
|
E: Bottles 17-20 |
750
|
750
|
50
|
F: Bottles 21-24 |
0
|
1500
|
50
|
Results
After nine weeks the bottles containing the compost material were emptied and their contents were examined to determine which of the composting methods tested was the most successful. Several additional questions were answered by analyzing the data that had been collected.
Periodic weight measurements indicated that the mass of compost material decreased substantially during the course of the experiment. Table 2 and figure 1 show the absolute mass of the contents of each compost treatment at various stages of the experiment. Table 3 and figure 2 displays the same data, converted into percentages of its initial value.
Table 2: Weight of compost material at certain time intervals: (expressed in grams)
blank |
Start |
1 Week |
4 Weeks |
6 Weeks |
9 Weeks |
Leaves only |
884.88g
|
595.05g
|
456.40g
|
362.40g
|
232.80g
|
Leaves & Food |
1565.88
|
944.58
|
622.19
|
384.17
|
203.90
|
Food Only |
1659.66
|
823.93
|
276.27
|
130.75
|
20.06
|
Leaves & Worms |
1337.06
|
1020.49
|
741.77
|
527.46
|
303.49
|
Leaves Food & Worms |
1640.76
|
1083.24
|
646.68
|
421.13
|
204.01
|
Food & Worms |
1989.99
|
742.01
|
356.55 |
248.33 |
59.56
|
Figure 1: Weight of compost material at certain time intervals: (expressed in grams)

Table 3: Weight of compost material at certain time intervals: (expressed as a percentage of initial weight)
blank |
Start |
1 Week |
4 Weeks |
6 Weeks |
9 Weeks |
Leaves only |
100.00%
|
67.25%
|
51.58%
|
40.96%
|
26.31%
|
Leaves & Food |
100.00
|
60.32
|
39.73
|
24.53
|
13.03
|
Food Only |
100.00
|
49.64
|
16.65
|
7.88
|
1.21
|
Leaves & Worms |
100.00
|
76.32
|
55.48
|
39.45
|
22.70
|
Leaves Food & Worms |
100.00
|
66.02
|
39.41
|
25.67
|
12.43
|
Food & Worms |
100.00
|
37.29
|
17.92
|
12.48
|
2.99
|
Figure 3: Weight of compost material at certain time intervals: (expressed as a percentage of initial weight)
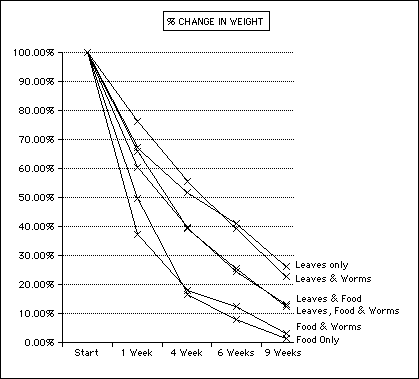
The weight of all six compost treatments decreased significantly during the course of the nine week experiment. The bins that lost the greatest percentage of their original weight were those that contained food. The leaf bins lost much less weight. ANOVA (Analysis of Variance) data proves that the large weight loss differences between the food treatments and the leaf treatments were significant, and that the smaller weight loss differences between the worm treatments and the no-worm treatments were insignificant.
The ANOVA data below verifies that the presence or absence of worms did not affect the weight of the compost treatments. Since P is 0.562, there is a 56.2 percent chance that any variance in weight the between worm and the no-worm treatments occurred purely by chance. Any percentage above 5 percent is generally considered to be too high. The ANOVA data shows that the presence of food versus leaves truly did affect the amount of decrease in compost weight. In this case the probability of error is zero.
Table 4: Analysis of Variance for Percent Weight Decrease
Source |
DF |
SS |
MS |
F |
P |
WormP/A |
2
|
0.000518
|
0.000518
|
0.35
|
0.562
|
Food/Lvs |
2
|
0.202297
|
0.101149
|
68.33
|
0.000
|
WormP/A*Food/Lvs |
2
|
0.002759
|
0.001380
|
0.93
|
0.412
|
Error |
18
|
0.026645
|
0.001480
|
blank |
blank |
Total |
23
|
0.232219
|
blank |
blank |
blank |
As expected, the bins containing both food and leaves lost less weight than the food-only bins, but more weight than the leaf-only bins. Weight loss occurred when material left through the top of the open soda bottles. To find out exactly what caused this loss or material, additional work had to be done. One possibility was that evaporating water caused the compost to lose weight. This possibility was tested by measuring the amount of moisture contained within the compost material before the experiment began, and again after the experiment had ended. It turned out that a considerable amount of moisture was lost from the compost material, particularly the food products. However, not enough moisture evaporated to fully account for the staggering amount of weight loss that occurred.
Table 5 shows the results of the test that found that the percentage of moisture in the compost ingredients, before and after the composting process. The initial moisture level within the leaves was found to be 51%, while the food products were found to be composed of nearly 97% water. After the composting process ended, the moisture levels of the leaves and the food had been reduced to 24.94% and 22.30%, respectively.
Table 5: The change in moisture of compost ingredients during a 9-week period.
blank |
Initial % Moisture |
After 9 weeks |
Leaves |
51.93%
|
12.95%
|
Food |
96.89
|
21.61
|
Moisture levels below 50% are generally too low for worms to survive. Table 6 indicates that the decreasing levels of water within the food partially explain the steadily decreasing weight of the food itself during the composting process. Figure 3 displays this trend more clearly with a graph.
Table 6: Change in the weight of leaves over a period of time as compared to the change in the weight of the water existing within those leaves over the same period.
blank |
Week 0 |
Week 1 |
Week 4 |
Week 6 |
Week 9 |
Weight of leaves |
884.88
|
595.05
|
456.40
|
362.40
|
232.85
|
Weight of water within leaves |
459.51
|
320.28
|
183.82
|
90.25
|
30.14
|
Figure 3: Change in the weight of leaves over a period of time as compared to the change in weight of the water existing within the leaves over the same period:
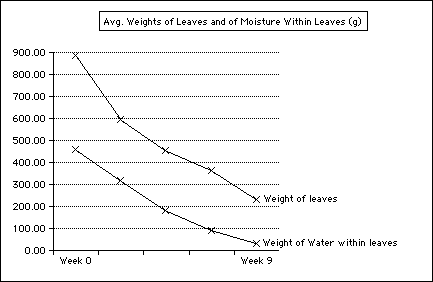
Similarly, the evaporation of water from the food products partially explained the weight lost from the food during the experiment. Table 7 displays this trend in chart form; figure 4 shows a graph of the same data.
Table 7: Change in the weight of food over a period of time as compared to the change in the weight of the water existing within the food over the same period:
blank |
Week 0 |
Week 1 |
Week 4 |
Week 6 |
Week 9 |
Weight of food |
1659.66
|
823.93
|
276.27
|
130.75
|
20.06
|
Weight of water within food |
1608.04
|
593.22
|
141.72
|
45.89
|
4.33
|
Figure 4: Change in the weight of food over a period of time as compared to the change in the weight of the water existing within that food over the same period:
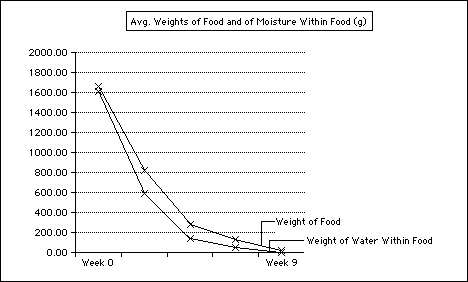
This data seems to indicate that the evaporation of water was an important cause of weight loss during the experiment. However, it also indicates that evaporation was not the only cause of weight loss. The previous two graphs show that the weight of the compost ingredients decreased slightly more rapidly than the weight of the water within those ingredients. Equidistant graph lines would have indicated that water evaporation was the only cause of weight loss. But it appears that if the experiment had been continued past its nine week duration, the graph lines would have eventually converged. The additional weight loss occurred when organic processes caused carbon dioxide to form and escape from the compost bottles.
Also observed was the depth of the contents of each compost bin. The bins started with between 6 and 8 inches of compost, with the deeper bins containing leaves, which are less dense, and the shallower bins containing food, which is more dense.. Tables 8 and 9 show the changing depths of the compost bins over time. Figures 5 and 6 illustrate these changes more clearly with graphs.
Table 8: Depth of compost material at certain time intervals: (expressed in inches)
blank |
Start |
1 Week |
4 Weeks |
6 Weeks |
9 Weeks |
Leaves only |
8.00 |
6.56
|
5.93
|
5.63
|
5.13
|
Leaves & Food |
7.00 |
4.81
|
4.13
|
3.37
|
3.19
|
Food Only |
6.00 |
3.43
|
1.50
|
1.06
|
0.56
|
Leaves & Worms |
8.00 |
6.50
|
5.75
|
5.00
|
4.31
|
Leaves Food & Worms |
7.00 |
5.00
|
3.68
|
3.25
|
2.62
|
Food & Worms |
6.00 |
2.50
|
1.56
|
1.50
|
0.81
|
Figure 5: Depth of compost material at certain time intervals: (expressed in inches)
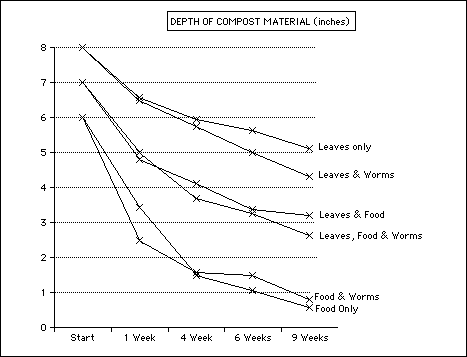
Table 9: Depth of compost materials at certain time intervals: (expressed as a percentage of initial depth)
blank |
Start |
1 Week |
4 Weeks |
6 Weeks |
9 Weeks |
Leaves only |
100% |
82.00%
|
74.21%
|
70.38%
|
64.12%
|
Leaves & Food |
100 |
68.71
|
58.93
|
48.29
|
45.57
|
Food Only |
100 |
57.28
|
25.00
|
17.67
|
9.33
|
Leaves & Worms |
100 |
81.25
|
73.44
|
62.50
|
53.88
|
Leaves Food & Worms |
100 |
71.43
|
52.67
|
46.43
|
37.57
|
Food & Worms |
100 |
41.67
|
26.04
|
25.00
|
13.50
|
Figure 6: Depth of compost materials at certain time intervals: (expressed as a percentage of initial depth)
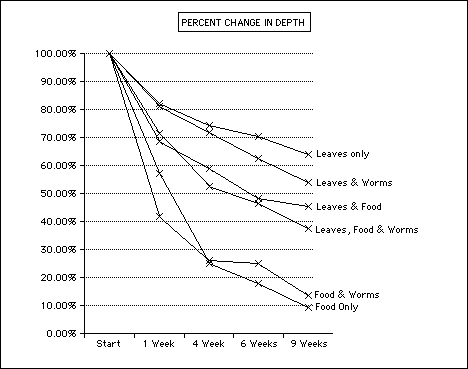
During the experiment, the depth of the compost material decreased substantially in each bin. The decrease in depth could have been caused by some of the same factors that explain the decrease in weight: evaporation of water and the emission of carbon dioxide. The bins containing food decreased in depth much more than those containing leaves. The food products quickly shriveled up and became unrecognizable. Meanwhile the leaves settled slightly and became crackly, but remained recognizable. As the above graph indicates, the presence of worms did not have a substantial impact upon the depth of the composts. The ANOVA data below seems to provide proof of the uselessness of worms in this experiment. Since P is 0.144, there is a relatively high chance that any variations between the treatments with worms and the treatments without worms occurred purely by chance. The data also proves that a major determinant of compost depth was whether the compost contained food or leaves.
Table 10: Analysis of Variance for Percent Decrease in Depth
Source |
DF |
SS |
MS |
F |
P |
WormPresance/Absence |
1
|
0.01311
|
0.01311
|
2.34
|
0.144
|
Food/Leaves |
2
|
0.92463
|
0.46232
|
82.41
|
0.000
|
WormP/A*Food/Lvs |
2
|
0.02390
|
0.01195
|
2.13
|
0.148
|
Error |
18
|
0.10098
|
0.01195
|
blank |
blank |
Total |
23
|
1.06263
|
blank |
blank |
blank |
In addition measuring to the weight and depth of the compost material, the density of the material was also measured. The density of the material in the compost bins reflected interesting relationships between the weight and the depth of the material, especially when observed over time. Table 11 displays the density of the material inside each compost treatment at different time intervals. Figure 7 depicts this data in a line graph. Table 12 and figure 8 express the changing densities of the material as a percentage of initial density. Table 13 provides ANOVA data showing the significant factors behind density change.
Table 11: Density of compost material at certain time intervals: (expressed in grams per inch of depth)
blank |
Start |
1 Week |
4 Weeks |
6 Weeks |
9 Weeks |
Leaves only |
110.61 |
90.92
|
76.93
|
64.15
|
45.37
|
Leaves & Food |
223.69 |
195.55
|
150.56
|
113.68
|
62.41
|
Food Only |
276.61 |
241.52
|
179.06
|
119.38
|
31.88
|
Leaves & Worms |
167.13 |
157.03
|
128.78
|
105.27
|
70.30
|
Leaves Food & Worms |
234.39 |
214.31
|
167.99
|
127.87
|
75.41
|
Food & Worms |
331.66 |
292.03
|
223.51
|
156.61
|
63.78
|
Figure 7: Density of compost material at certain time intervals: (expressed in grams per inch of depth:
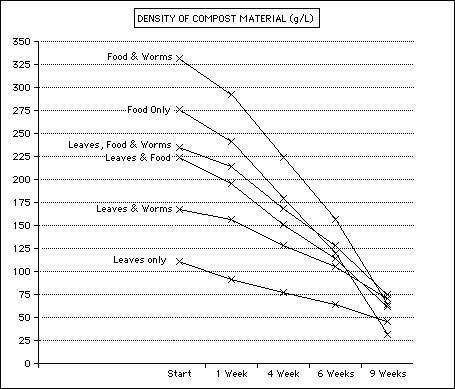
Table 12: Density of compost materials at certain time intervals: (expressed as a percentage of initial density)
blank |
Start |
1 Week |
4 Weeks |
6 Weeks |
9 Weeks |
Leaves only |
100% |
82.20%
|
69.55%
|
58.00%
|
41.02%
|
Leaves & Food |
100 |
87.42
|
67.31
|
50.82
|
27.90
|
Food Only |
100 |
87.32
|
64.74
|
43.16
|
11.53
|
Leaves & Worms |
100 |
93.96
|
77.06
|
62.99
|
42.06
|
Leaves Food & Worms |
100 |
91.43
|
71.67
|
54.56
|
32.17
|
Food & Worms |
100 |
88.05
|
67.39
|
47.22
|
19.23
|
Figure 8: Density of compost materials at certain time intervals: (expressed as a percentage of initial density)
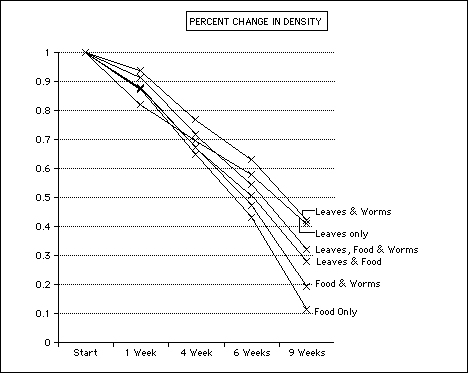
Table 13: Analysis of Variance for Density
Source |
DF |
SS |
MS |
F |
P |
WormPresance/Absence |
1
|
0.010496
|
0.010496
|
1.65
|
0.215
|
Food/Leaves |
2
|
0.286779
|
0.143390
|
22.58
|
0.000
|
WormP/A*Food/Lvs |
2
|
0.004805
|
0.002403
|
0.38
|
0.690
|
Error |
18
|
0.114322
|
0.006351
|
blank |
blank |
Total |
23
|
0.416402
|
blank |
blank |
blank |
The density of the material within each compost treatment decreased substantially throughout the experiment. The compost bins containing leaves generally decreased in density more slowly than those containing food. This decrease in density indicates that although the volume of material steadily decreased during the experiment, it did not decrease enough to offset the decrease in the weight of the compost material. In other words, moisture and carbon dioxide left the compost (reducing the weight), and as the amount of weight decreased, less moisture remained to press the compost into a tighter, more dense heap. The resulting compost was a fairly compressed, dry heap of material that had lost considerable volume, but enough volume was lost to make up for the considerable loss in weight.
All in all, the presence of worms seemed to have the effect of increasing the densities of the compost materials, but not be a statistically meaningful amount. As the above ANOVA data shows, there is a 21.5% probability that random factors were behind this increase in density. The apparently increased densities of the compost material containing worms could have resulted from the worms' squirming around and causing the contents of the bins to settle during the compost process, decreasing the volume and shifting the weight to volume ratio. Another explanation: the worms consumed water, and the water that was inside the worms' bodies was protected from evaporation. The majority of the worms died however, and when they shriveled up and dried out, the water that had been inside them was free to evaporate. Therefore if the worms had any affect at all upon the density of the compost material at all, it was probably because they caused the material to settle.
Discussion
Several concerns were brought up while this experiment was in the planning stages. Most of these had to do with reducing the variety of unpredictable external forces that could affect the compost treatments differently. The first was that each of the compost treatments had to be aerated equally. The bottles containing food were expected be more dense and more moist than those containing leaves. In order to space out the food chunks and to keep an adequate amount of air flowing through the food bins, Styrofoam packaging peanuts were to be combined with the compost. However it was later determined that this would have contributed to an unrealistic composting environment which would have had no application in the real world. Compost combined with non-biodegradable Styrofoam peanuts would be of no value in an agricultural setting. Furthermore, the effect of Styrofoam on worms was unknown. The Styrofoam idea was abandoned before the experiment began.
Keeping all compost treatments equally moist was another concern. In order to keep the compost from becoming too moist, small air holes were poked in the sides of the soda bottles. The holes were meant not only to keep the composts from becoming too moist, but also to supply the worms with enough air to survive. It was also important that each of the compost treatments was exposed to the same lighting conditions. If some bottles had been in the shade and others in direct sunlight, conditions within them would not have been consistent. A shady counter top in a greenhouse was used to house the compost bottles. Periodically the bottles were randomly rearranged to ensure that no bottle was placed in what could have been an overly shady or bright spot for any longer than any of the other bottles. Similarly, the bottles were rearranged to make sure that no one bottle was exposed to more heat than any of the others. Finally, a constant ambient temperature was maintained within the greenhouse to ensure that conditions remained consistent for each compost treatment.
Several additional questions and concerns came up while the experiment was being planned and executed. Many of these concerns led to eventual changes in the experimental design. For example, it was impossible to weigh an entire compost bin without overloading the precise scale that was being used. The solution was to weigh 1.0 L of the compost at a time, instead of weighing the full 2.0 L soda bottle. This seemingly acceptable solution created yet another problem: when a 1.0 L volume of compost was weighted, it was actually not the weight but the density of the compost that was in effect being measured. This error was not realized until late in the data analysis process. As a result, a lot of work had to be redone. When these false weight measurements were eventually found to be useful as density measurements, the correct weights were extrapolated from two sets of numbers that were known to be correct: the depth readings and the newfound density measurements.
On a different note, it was decided that the compost treatments that needed to include include worms were to be given 50 g of worms. It was expected that this quantity of worms would thrive in a 2 Liter environment. Early on in the execution of the experiment, it seemed as though the worms might not have enough water to survive. Each of the bottles that contained pure leaves received 40 mL of tap water to keep the bottles moist enough to support life. Each of the bottles that contained a mixture of leaves and food received 20 mL of water to keep the bottles moist enough to support life. Each of the bottles that contained only food received no additional water; the environment inside the bottles was presumably already moist enough to support the worms. Bottles received water regardless of weather they contained worms or not, keeping the number of variables to a minimum. Another problem that developed early in the experiment was the formation of mold inside the compost bottles- particularly on the surface of the compost heaps, just under the plastic seal covering the tops of the bottles. This mold may have been interfering with the decomposition of the food and leaves. At the time it was unknown what effect the mold would have on the worms. The mold was accompanied by a pungent smell however, which could not have been very healthy for the worms. The smell that accompanied the mold indicated that an anaerobic fermentation process was occurring within the compost bottles. Since anaerobic processes can only occur in environments free of oxygen, this should have served as a warning that there was not much oxygen available for the worms to breathe.
Before long many of the worms died. Their death was initially blamed on an overabundance of moisture inside the bottles. Closer observation revealed that the true cause of the worms' suffering was multifaceted. A major cause was a lack of oxygen and a corresponding overabundance of carbon dioxide inside the bottles. Despite their natural aversion to sunlight, many of the worms attempted to leave the confines of the soda bottles by crawling to the top of the compost piles and squeezing past the rubber band holding down the plastic seal. The worms were suffocating under the air-tight plastic wrap that covered the top of each compost bottle. These plastic seals were removed after four days. It was estimated that almost half of the worms asphyxiated. 20 grams of worms were added to each of the twelve bottles in treatments D, E, and F, to make up for those that had been lost. Once the plastic wrap covers were removed and worms were replaced, none of the new worms attempted to escape from the bottles. Most of the mold also disappeared shortly after the plastic was removed, and a dryer, more oxygen rich environment had been restored.. The smell that had accompanied the mold also dissipated somewhat at this time, indicating that an aerobic composting environment had been restored. Volatile organic acids (VOAs) are expected to have been the cause of the odor problem. VOAs are heavier than air and can sometimes linger around the site they originate from for a long time, instead of dissipating. A BioCycle article sheds some more light upon the conditions that can lead to odor problems:
Most of the underlying causes [of odor problems] lead to the development and persistence of anaerobic conditions and consequently VOAs. Therefore, many of the underlying causes of odor relate to poor air penetration (high bulk density, high moisture, small particles). In many cases, the cause is either excessive moisture or a mix of materials that doesn't provide enough porosity and structure, even at acceptable moisture levels. Such a mix would tend to be heavy (high bulk density). Odors from anaerobic conditions also occur with materials that decompose rapidly, that use oxygen faster than it can be resupplied. (Thomas, 1999.)
The composts in this experiment had many of the characteristics mentioned above, particularly high bulk density and high moisture. These factors probably would have prevented enough oxygen from entering the compost bottles early in the experiment- even if the bottles themselves had not been covered by plastic wrap.
Once the plastic seals were removed from the bottles, the worms seemed to thrive. The bins that contained a mixture of food and leaves seemed to be the best environment for the worms. The bins containing only food however were still too moist and dense. The worms in the bins containing only leaves did not move around much, since they could not burrow through the loose leaves very easily They tended to remain in a tangled clump in the middle of the mass of leaves. Since they could not move around and eat they were the first to die off. The worms in the bins containing only food died off next. Since the contents of the food bins settled considerably early on in the experiment, the worms did not have much space. In addition, their environment was still very moist and moldy, and once the food rotted or was consumed, the worms starved.
If the worms had been given a frequently-replenished supply of food they probably would have survived. The fact that worms consume huge quantities of food was unfortunately overlooked. The worms' living conditions prior to the experiment should have indicated that the amount of food that they would need was huge: before they were put in the compost bins, the worms were kept in a cat litter box filled with strips of paper. The two pounds of worms that were being kept inside the box ate all the paper within a week or two. The box was refilled twice, only to be emptied again by the voracious worms. Despite this advance warning, the worms' food supply was never replenished once the experiment began. The justification for not replacing the worms' food supply was that replacing the food would have thrown off the mass and depth measurements. Since the worms died off early on in the experiment, most of the decomposition was accomplished by microbes.
Another factor that may have contributed to the demise of the worms was the excessively high temperature inside the compost bins. The temperature was high enough to melt the worms. Interestingly, some of the conditions within the compost bottles seemed to suggest that the temperatures were actually not as high as indicated by the worms' melted bodies. For example, the volume of each compost was smaller than that which is normally required for the buildup of heat. In addition, the compost environments were anaerobic early on in the process, meaning that the temperature inside each bottle probably remained fairly low. Furthermore, it is possible that the worms melted because of excess moisture, rather than excess heat. But even if temperature was not a factor in the worms' death, any combination of the other possible causes could certainly have been harmful enough to kill the worms.
Another concern was that excessive moisture might escape from the compost bins during the experiment, leaving the bins too dry to support worm life. Therefore it seemed important to collect data on moisture. The amount of moisture within the compost ingredients was determined before any decomposition had taken place. After the experiment concluded, the amount of moisture within the compost was again measured. These measurements were used to determine how much moisture escaped from the compost during the nine week experiment. A moisture level of 50% is considered adequate. (Thomas, 1999)
Towards the end of the experiment it became evident that the compost treatments containing a mixture of leaves and food were retaining a lot of moisture, while the compost bottles containing either pure leaves or pure food each retained very little moisture. The cause for this was unknown. It was assumed that within the bottles containing both leaves and food, the leaves created a barrier that did not allow moisture to escape from the food. However this answer did not explain why the leaves alone lost so much moisture. Perhaps the leaves, when not combined with food, lost much of their moisture because they were not packed down nearly as tightly as when they were mixed with comparatively heavy food. The leaves alone were less dense and more exposed to air circulation, which may have allowed the moisture within and around them to evaporate.
The food bottles started off just as moist if not more moist than the bottles containing both leaves and food. Therefore it might seem surprising that the compost treatments containing only food lost much more moisture than the bottles containing a mixture of food and leaves. The reason the food bottles lost so much moisture seems to be twofold. First, the food did not have a protective leaf layer to retain moisture, and second, since the food itself disappeared during the course of the experiment (losing over eighty percent of its volume in the process) eventually an inadequate quantity of compost material remained to prevent even more moisture from evaporating.
One final problem that became noticeable shortly after the bottles were uncovered was that a great deal of compost material was disappearing. It was not known why initially, but it seemed that the only place the material could have gone was into the air outside the compost bottles. It seemed possible that bugs ate some of the compost, but very few insects lingered around the compost treatments once the bottles had been left uncovered for a few days. This loss of compost material remained inexplicable until long after the experiment had ended, while data was being analyzed. In the meantime, a new question was raised: would any compost material have escaped if the bottles had remained sealed all along?
Conclusions and Reflection
Once the collected data was analyzed, it became possible to answer most of the questions posed at the beginning of this paper. The first question asked which of the six compost methods tested is most successful at producing humus (fertile compost.) The answer is based on the appearance and texture of the finished compost material.
The contents of compost treatment C, which contained food only, rather closely resembled soil after the nine week experiment had ended. The following table shows a rundown of the rest of the results:
Table 14: Decomposition Results:
Most decomposed |
Compost Treatment C (Food only) |
Second most decomposed |
Compost Treatment F (Food and worms) |
Third most decomposed |
Compost Treatment B (Leaves and food) |
Fourth most decomposed |
Compost Treatment E (Leaves, food, and worms) |
Fifth most decomposed |
Compost Treatment D (Leaves and worms) |
Least decomposed |
Compost Treatment A (Leaves only) |
The compost treatments containing food decayed more completely than the treatments containing leaves. Food was an ingredient in the four most decomposed treatments, while leaves were found to have been in the four least decomposed treatments. Clearly food is a more effective ingredient than leaves when it comes to creating a thoroughly decomposed compost in a limited amount of time. Compost treatments containing worms do not appear to have decomposed more thoroughly than those not containing worms. Since worms were included in the second, fourth, and fifth most decomposed treatments, it can be concluded that worms had a slightly negative impact upon the decomposition process. If the most decomposed compost treatment had contained worms, it would have been possible to claim that worms had a slightly positive effect upon the decomposition process.
Although compost treatment C had entered an advanced state of decomposition by the end of the experiment, it did not appear quite ready to be used as soil in a garden. There are certain drawbacks to using compost that has not been given enough time to fully decompose. According to BioCycle:
Failure to produce a mature compost product -- why is that a problem? Depending on when and how it is used, an immature compost can have qualities that hurt its performance and value. Immature composts may contain high levels of VOAs, ammonia, and readily available carbon. Both ammonia and VOAs are harmful to plants, especially seedlings. Furthermore, high ammonia levels can lead to nitrogen loss. An immature compost can also tie up or immobilize nitrogen. The C contained in immature compost is still easily available, and in the process of decomposing that C, microorganisms also use the available N from the compost and surrounding soil. This is more likely to occur in compost as t the C:N ratio increases (from 30:1 up) (Thomas, 1999)
The next question asked if food scraps decompose more quickly than leaves. Table 14 reveals that the answer is a definite yes. At the end of the nine week experiment the food scraps were completely unrecognizable, while most of the leaves were clearly identifiable. Furthermore, it was found that the decomposition rate of leaves can be increased with the addition of food. This is true because the leaves in treatments A and D ended up in a much more recognizable state than the leaves in treatments B and E. (A and D contained leaves only, while B and E contained a mixture of leaves and food.)
The next question asked if the presence of worms affected the decomposition process. It was found the presence of worms did not affect the decomposition process substantially. This is indicated by the ANOVA data found in Table 4, which shows that P is 0.562. Therefore there is too great a probability (56.2%) that the recorded differences in weight between compost treatments containing worms and those not containing worms are due to random occurrences rather than substantial differences between having worms and not having worms in a compost.
In a similar analysis, Table 10 shows that worms also had no significant affect upon the depth of the composts. There is a 14.4% probability that the recorded differences in depth between compost treatments containing worms and those not containing worms are due to chance. Therefore it can not be assumed that the presence of worms had any effect upon the depth of the composts.
Finally, it appears that worms had no effect upon the density of the compost material. According to Table 13, there is a 21.5 percent chance that worms had no effect upon the density of the compost. The conclusion that worms accomplished nothing in this experiment is based on incomplete evidence; most of the worms died long before the completion of the experiment. If the worms had lived throughout the experiment it would have been possible to determine whether they had an impact upon the decomposition rates of the food and leaves.
Although the worms did not thrive under any circumstances, they survived slightly longer in the presence of rotting food than in the presence of rotting leaves. The best environment for the worms, from among the three tested, seemed to be a mixture of food and leaves. It is expected that under the right conditions, the worms would have had a significantly greater impact upon the decomposition process. The Dakota Blue Worm Farm maintains a Web site that provides valuable information on exactly how to keep worms alive and well. The site provides several rules of thumb that were clearly violated while this experiment was being conducted. First, the site suggests using a sheltered location that gets some sun, but not so much sun that the composts dry out and become too hot. Since the plastic soda bottles used in this experiment were transparent and in direct sunlight most of the time, they were allowed to become too hot and dry. Next the site explains how to properly feed the worms:
The recommended ratio of worms to food is: For about 400 grams per day of food waste (a typical amount for a family of four to six), use a minimum of 500 grams of worms (just over 1000 worms) and preferably 900 grams (about 2000 worms). It doesn't matter if you are unable to obtain so many worms initially - just reduce the quantity of food fed accordingly. If you provide ideal conditions, the worm population will steadily increase, doubling their numbers every 30-60 days. (Thomas, 1999.)
For this experiment, the number of worms was not gradually built up over time. Instead the entire population of worms was present in the compost at the beginning of the experiment. Another mistake was that instead of continually adding food over time, no additional food was ever added to the compost. The site goes on to explain that worms require a comfortable bedding in which to live and lay eggs:
Suitable materials [for a bedding] include shredded and moistened newspaper (avoid colored print, and tear newsprint into strips) as well as cardboard, peat, straw, and aged manure or compost. Other materials such as old grass clippings and other aged brown plant debris can be used, but it is IMPERATIVE that no green materials or fresh manure be added. These materials will heat up and kill the worms. Sawdust is not recommended due to its resin content. The addition of one or two handfuls of coarse sand or top soil will provide the grit that the worms need to grind their food. The worm bin should be three quarters full with a damp bedding that has a consistency of a squeezed out sponge. It must be moist but not soggy. Soak overnight to wet bedding if necessary, allowing time to drain or squeeze out the excess moisture. Gently place bedding in position avoiding compaction, as air spaces are necessary for successful composting by helping to prevent odors and allowing easier movement and air for the worms. (Thomas, 1999.)
Obviously the worms used in this experiment suffered from a complete lack of bedding. They were given only course dead leaves and overly moist rotting food to live in. These materials were far too hot, too compact, and too full of harmful odors. Finally, the site reiterates a few helpful suggestions, many of which were violated in this experiment:
Kitchen food waste has a high moisture content. To prevent sour slimy conditions from developing, creating odors and attracting flies, add food regularly rather than in large quantities. Grinding in a food processor is also helpful. Add a little crushed egg shells helps add needed calcium to the bed. It may also be necessary to sprinkle a SMALL amount of garden lime on the beds every now and then to reduce acid in the bed. Worms don't like acidic conditions. Worms need to be kept moist but not soggy. If they dry out they will die, or if it's too wet they will drown. Fruit and house flies can be troublesome if added food isn't buried in the bedding. If odors are produced, this is usually because the bin has been overfed, allowed to become to moist or else the bedding has become packed, limiting air flow. Odors result from anaerobic (lack of free oxygen)
conditions, so it is important to correct not only for odor reasons but also because the conditions may well be toxic to the worms which need oxygen to live. As worm composting doesn't generate heat, any seeds included with the food may still germinate. (Thomas, 1999.)
Had the above information been known prior to the execution of this experiment, it would have been easier to confirm or deny the benefits of worms upon compost. The results of a BioCycle study entitled A Comparison of Vermicomposting and Composting indicate that worms, when treated properly, do indeed provide great benefits to the compost pile. The study's conclusion reads:
Preliminary research in our laboratory showed that vermicomposting involves great reduction in populations of pathogenic microorganisms, thus not differing from composting from this point of view. Generally it is accepted that the thermophilic stage during the composting process eliminates the pathogen organisms, but we have shown that pathogens are eliminated during vermicomposting. As an aerobic process, composting leads to a nitrogen mineralization and the use of earthworms in vermicomposting increases and accelerates this nitrogen mineralization rate. The humification processes that take place during the maturation stage of composting are greater and faster during vermicomposting. Vermicomposting may also bring about a greater decrease of bioavailable heavy metals than in the composting process, and there is evidence that the final product may contain hormone like compounds which accelerate plant growth. (Dominguez, 1997.)
The conclusion of the BioCycle study also explains that as the number of worms used increased, the net content of nitrogen in the compost decreased. Worms also successfully reduced the toxicity of the compost mixtures. Finally, it was confirmed that worms destroyed many types of pathogens, including those found in human waste.
This conclusion definitively answers one of the questions that this experiment failed to answer: Do worms speed up the decomposition process? The answer is yes. Although the BioCycle study does not provide any insight on some of the other questions addressed in this experiment, (chiefly the effects of worms upon weight, depth, and density of compost material) it does provide significant evidence that worms affect the more important aspects of compost: the speed of the decomposition process and the quality of the final product.
The next question that was asked in the beginning of this report concerned the difference between the quantity of the input (food and leaves) and the quantity of output (fertile compost.) It was found that a very large amount of weight was lost from the compost bins during the experiment. This loss in weight can be attributed to both the evaporation of water and the emission of carbon dioxide. The ANOVA data in Table 4 indicates that the amount of weight lost from the composts was highly dependent upon whether the compost contained food or leaves.
The next question asked: Would the contents of the compost bins settle during the composting process? The answer is yes. The contents of each compost bin settled substantially. The food however, settled significantly more than the leaves, as shown in Table 10.
Additional Considerations
Although this experiment produced a large amount of useful information, several changes in the experimental design would have been beneficial. First, the living conditions for the worms should have been improved. Providing the worms with a habitable environment, with plenty of space, food, oxygen, soil, and no harmful odors, would have led to a discovery of what compost materials are most suited for worms. The continuous addition of food however, would have made accurate measurements of weight and depth changes extremely difficult. The search for the best compost environment for worms may have been better conducted as a separate experiment, one in which the changes in weight and volume of the compost itself was not a concern. The inevitable benefit of this flawed experiment is that it is now known exactly what kind of environment is inhospitable to worms.
Another improvement in the experimental design might have been the use of larger compost bins. These would have allowed for more natural processes to occur, such as the heating of the compost pile. A larger compost would also have prevented the volume of compost material from being reduced to a negligible amount. In this experiment, only half an inch of compost material remained in what had been a 6-inch pile of food nine weeks earlier.
Additionally, weight and depth measurements should have been taken more frequently and at more regular intervals. Although measuring the weight and depth of each compost bottle five times during the course of the experiment proved to be enough to extract meaningful information, it would have been desirable to have more information to draw from when graphing trends such as weight loss and density change.
Yet another improvement in the experimental design would have been the use of an exclusively aerobic composting environment instead of an anaerobic composting environment. During this experiment the composting environment quickly changed from an aerobic one to an anaerobic one, and then back again. The environment should have remained consistent throughout the experiment. The unintended effects of using plastic wrap to cover the compost bottles included the suffocation of most of the worms, the emission of a putrid odor caused by anaerobic microbes, and the growth of mold.
Also, temperature changes should have been recorded at regular intervals throughout the experiment. The temperature within the compost bottles should have been recorded because it would have helped to determine whether the temperature was getting too high for the worms. Too low a temperature however, would have been indicative of anaerobic fermentation, which was also undesirable. Knowing the temperature would have made it easier to guess how well the decomposition process was preceding.
Finally, more preliminary research should have been conducted before this experiment was designed and executed. Specifically, had I known more about the conditions that are necessary for the survival of worms, this experiment would have been more successful. As it turned out, the necessary research was done, but only after certain failures made it necessary to find out what had gone wrong. Despite its failings, this experiment was a success in that it led to the discovery of a large body of composting knowledge.
References
Acheson, Katherine et. al. Disposing of the Organic Wastes Produced by the Food
Services of Brown University (Novemver 1995) http://www.brown.edu/
Departments/Brown_Is_Green/esproj/owst1195/>
The Digital Composter: What is compost? (February 25, 1999) <http://www.digitalseed.
com/composter/whatiscompost.html>.
Dominguez, J. et al. "A Comparison of Vermicomposting and Composting"
BioCycle (April 1997): 57-59.
Eastman, Bruce R. "Achiving Pathogen Stabilization using Vermicomposting"
BioCycle (Novemver 1999): 62.
Gotaas, Harold, Composting. Geneva: World Health Organization, 1956,
Minnich, J. et al, "The Rodale Guide to Composting", (Emmaus, Pennsylvania:
Rodale Press, 1979)
Seekins, Bill "Troubleshooting The Compost Pile" BioCycle (November 1999): 53.
Thomas, P. Quick Start Guide to Worm Composting, Dakota Blue Worms Company Web
Site. <http://home.isoa.net/~pthomas/castings.html>